mboost-dp1
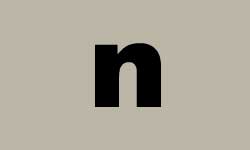
MIT
- Forside
- ⟨
- Forum
- ⟨
- Nyheder
#49
Du udsættes for mere radioaktiv stråling ved at sove ved siden af en person, end du gør ved at bo i nærheden af et A kraftværk.
#50
Nej, Akraftværker idag er bygget med passiv beskyttelse mod sådanne ting.
http://en.wikipedia.org/wiki/Passive_nuclear_safet...
Du udsættes for mere radioaktiv stråling ved at sove ved siden af en person, end du gør ved at bo i nærheden af et A kraftværk.
#50
Nej, Akraftværker idag er bygget med passiv beskyttelse mod sådanne ting.
http://en.wikipedia.org/wiki/Passive_nuclear_safet...
Porre: Ja, de nyeste værker... Men du skrev "kan ikke ske i dag", hvilket var hvad slog mig... Langt de fleste reaktorer der er i drift, er af typer hvor du godt kan lave noget tåbeligt hvis du aktivt forsøger det...
Men selv om der er passiv beskyttelse, så er der stadig mulighed for at få lortet til at gå galt, det er et spørgsmål om en usund kombination af ihærdighed og dumhed >_<
Men selv om der er passiv beskyttelse, så er der stadig mulighed for at få lortet til at gå galt, det er et spørgsmål om en usund kombination af ihærdighed og dumhed >_<
Der kommer strengere og strengere regler og opsyn på værkerne, især efter fx fuckshima incident, men jo der findes mange ældre værker, især når vi drager østpå. De skal bare skiftes ud.
Men i snakken om at få mere akraft så må man gå ud fra at de ikke bygger et 30 år gammelt kraftværk når der skal nyt til ^^
Jo før vi kommer af med kul jo bedre.
Det er den billigste, og sundeste kilde til strøm, også når man tager waste i med i beregningen. Jeg vil hellere have det radioaktive materiale i en bøtte i et kontrolleret område, frem for i luften som det er tilfældet med kul.
Men i snakken om at få mere akraft så må man gå ud fra at de ikke bygger et 30 år gammelt kraftværk når der skal nyt til ^^
Jo før vi kommer af med kul jo bedre.
Det er den billigste, og sundeste kilde til strøm, også når man tager waste i med i beregningen. Jeg vil hellere have det radioaktive materiale i en bøtte i et kontrolleret område, frem for i luften som det er tilfældet med kul.
Det er fuldt ud muligt at lave en reaktor, der ikke nedsmelter, selvom alle strømkilde svigter (eksterne, turbine, dieselgeneratorer etc.). Om de holder til en tsunami ved jeg ikke.
"Integral Fast Reactor", men kloge demokrater i USA valgte, at de ikke ville understøtte udviklingen af en reaktor, hvis brændselsstave yderligere var på baggrundstrålingsniveau efter 800 år og ikke 10000.
Hele antiatomtingene er noget værre bæ, der har holdt en ellers positiv udviklingen tilbage eller helt stoppet den. Delvist betalt for af olieinteressenter kan man undre sig en del.
"Integral Fast Reactor", men kloge demokrater i USA valgte, at de ikke ville understøtte udviklingen af en reaktor, hvis brændselsstave yderligere var på baggrundstrålingsniveau efter 800 år og ikke 10000.
Hele antiatomtingene er noget værre bæ, der har holdt en ellers positiv udviklingen tilbage eller helt stoppet den. Delvist betalt for af olieinteressenter kan man undre sig en del.
http://en.wikipedia.org/wiki/Integral_fast_reactor skrev:Advantages
Breeder reactors (such as the IFR) could in principle extract almost all of the energy contained in uranium or thorium, decreasing fuel requirements by nearly two orders of magnitude compared to traditional once-through reactors, which extract less than 0.65% of the energy in mined uranium, and less than 5% of the enriched uranium with which they are fueled. This could greatly dampen concern about fuel supply or energy used in mining. In fact, seawater uranium extraction could provide enough fuel for breeder reactors to satisfy our energy needs indefinitely, thus making nuclear energy as sustainable as solar or wind renewable energy.[4]
Breeder reactors can “burn” long lasting nuclear waste components (actinides: reactor-grade plutonium and minor actinides), turning liability into an asset. Another major waste component, fission products, would stabilize at a lower level of radioactivity in a few centuries, rather than tens of thousands of years. The fact that 4th generation reactors are being designed to use the waste from 3rd generation plants could change the nuclear story fundamentally—potentially making the combination of 3rd and 4th generation plants a more attractive energy option than 3rd generation by itself would have been, both from the perspective of waste management and energy security.
The use of a medium-scale reprocessing facility onsite, and the use of pyroprocessing rather than aqueous reprocessing, is claimed to eliminate any proliferation risk from possible diversion of fissile material.
Safety
In traditional light water reactors (LWRs) the core must be maintained at a high pressure to keep the water liquid at high temperatures. In contrast, since the IFR is a liquid metal cooled reactor, the core could operate at close to ambient pressure, dramatically reducing the danger of a loss-of-coolant accident. The entire reactor core, heat exchangers and primary cooling pumps are immersed in a pool of liquid sodium or lead, making a loss of primary coolant extremely unlikely. The coolant loops are designed to allow for cooling through natural convection, meaning that in the case of a power loss or unexpected reactor shutdown, the heat from the reactor core would be sufficient to keep the coolant circulating even if the primary cooling pumps were to fail.
The IFR also has passive safety advantages as compared with conventional LWRs. The fuel and cladding are designed such that when they expand due to increased temperatures, more neutrons would be able to escape the core, thus reducing the rate of the fission chain reaction. In other words, an increase in the core temperature will act as a feedback mechanism that decreases the core power. This attribute is known as a negative temperature coefficient of reactivity. Most LWRs also have negative reactivity coefficients; however, in an IFR, this effect is strong enough to stop the reactor from reaching core damage without external action from operators or safety systems. This was demonstrated in a series of safety tests on the prototype. Pete Planchon, the engineer who conducted the tests for an international audience quipped "Back in 1986, we actually gave a small [20 MWe] prototype advanced fast reactor a couple of chances to melt down. It politely refused both times."[5]
Liquid sodium presents safety problems because it ignites spontaneously on contact with air and can cause explosions on contact with water. This was the case at the Monju Nuclear Power Plant in a 1995 accident and fire. To reduce the risk of explosions following a leak of water from the steam turbines, the IFR design (as with other sodium-cooled fast reactors) includes an intermediate liquid-metal coolant loop between the reactor and the steam turbines. The purpose of this loop is to ensure that any explosion following accidental mixing of sodium and turbine water would be limited to the secondary heat exchanger and not pose a risk to the reactor itself. Alternative designs use lead instead of sodium as the primary coolant.
Efficiency and fuel cycle[edit]
The goals of the IFR project were to increase the efficiency of uranium usage by breeding plutonium and eliminating the need for transuranic isotopes ever to leave the site. The reactor was an unmoderated design running on fast neutrons, designed to allow any transuranic isotope to be consumed (and in some cases used as fuel).
Compared to current light-water reactors with a once-through fuel cycle that induces fission (and derives energy) from less than 1% of the uranium found in nature, a breeder reactor like the IFR has a very efficient (99.5% of uranium undergoes fission[citation needed]) fuel cycle.[6] The basic scheme used pyroelectric separation, a common method in other metallurgical processes, to remove transuranics and actinides from the wastes and concentrate them. These concentrated fuels were then reformed, on site, into new fuel elements.
The available fuel metals were never separated from the plutonium, and therefore relatively difficult to use in nuclear weapons. Also, plutonium never had to leave the site, and thus was far less open to unauthorized diversion.
Another important benefit of removing the long half-life transuranics from the waste cycle is that the remaining waste becomes a much shorter-term hazard. After the actinides (reprocessed uranium, plutonium, and minor actinides) are recycled, the remaining radioactive waste isotopes are fission products, with half-life of 90 years (Sm-151) or less or 211,100 years (Tc-99) and more; plus any activation products from the non-fuel reactor components. (Tc-99 and Iodine-129 are also candidates for nuclear transmutation to stable isotopes by neutron capture.)
Opret dig som bruger i dag
Det er gratis, og du binder dig ikke til noget.
Når du er oprettet som bruger, får du adgang til en lang række af sidens andre muligheder, såsom at udforme siden efter eget ønske og deltage i diskussionerne.